
Research
How do plants sense their
surroundings and respond?
In the Woodson lab, we are studying two major strategies plans use to match their physiology with the environment
Local intracellular signals controlling gene expression and cellular degrdation in reponse to stress
Rapid long-distance signals that communicate the stress status to disal tissues in order to promote stress resilienece
1. Chloroplasts signal to the nucleus to coordinate gene expression, maximize photosynthesis, and avoid stress.
As sessile organisms, plants must employ a wide range of signaling mechanisms to thrive in an ever changing environment. During photosynthesis, chloroplasts constantly experience metabolic stress in the form of reactive oxygen species (ROS). The severity, timing, and location of these stresses fluctuates with the environment, therefore chloroplasts are able to sense these changes and signal out for acclimation. In response to changing environments, chloroplasts can emit “retrograde” signals that alter gene expression and/or cell physiology. In some cases, chloroplast stress can be some extreme that individual chloroplasts are targeted by the cell for turnover. This is illustrated above in the transmission electron microscopy (TEM) image of Arabidopsis thaliana cells with green-colored chloroplasts. The chloroplast on the top left has suffered so much damage that it is being moved into the central vacuole for turnover. Such a chloroplast quality control pathway may be very important for plants to maintain healthy populations of chloroplasts performing efficient photosynthesis.
But what if only a few chloroplasts are stressed or damaged? A chloroplast quality control pathway would be needed to turnover and/or repair damaged organelles
Classic retrograde signals would not allow the nucleus to distinguish between damaged and healthy chloroplasts. Ignoring the problem would leave chloroplasts that are energetically inefficient, as well as dangerous ROS generators. Therefore, a chloroplast quality control mechanism could be used to repair or remove defective/stressed chloroplasts from overall healthy cells.
Open questions we are trying to answer:
How do organelles communicate with the cell?
How is chloroplast turnover/repair regulated?
Can we control how plants grow in stressful environments?
Evidence for a chloroplast quality control mechanism was first revealed in the Arabidopsis thaliana plastid ferrochelatase 2 (fc2) mutant. fc2 mutants cannot tolerate dark-to-light shifts due to a burst of singlet oxygen (1O2) attributable to the accumulation of the photosensitizing tetrapyrrole intermediate protoporphyrin-IX (Proto). The 1O2 burst leads to the ubiquitination of chloroplast envelope proteins, induction or chloroplast degradation, and eventually cell death.
The Woodson lab is currently interested in resolving three crucial questions about the chloroplast quality control mechanism(s):
1. Understanding the source of the stress signal; What triggers the chloroplast degradation pathway?
2. How is the signal transmitted? How can chloroplasts be targeted for degradation or repair?
3. What is the role of chloroplast quality control under natural stress conditions?
We have been supported by the Division of Chemical Sciences, Geosciences, and Biosciences, Office of Basic Energy Sciences, US Department of Energy (2018-2025, DE-SC0019573)
How is information communicated through the plant to distal tissues?
2. Stressed cells can also send information to distal tissues that control stress responses on the other side of the plant
The field of plant biology has mainly focused on how cells directly respond to stress and lead to local acclimation responses. However, stressed cells can also send rapid signals to other tissues to control stress responses. Often, these types of signals involve “waves” of metabolites such as reactive oxygen species (ROS), calcium ions (Ca2+), electrical and hydraulic signals. For instance, a leaf wound can lead to a Ca2+ wave that rapidly moves to other leaves within minutes. This can be visualized with genetically encoded Ca2+ sensors as shown above. However, how these signals are first initiated and ultimately control distal responses is not well understood.
We are currently part of an NSF Science and Technology Center (STC) called CROPPS (Center for Research of Programmable Plant Systems). One of our major goals is to use these long-distance waves to enable bi-directional communication with plants. For plant-to-person communication, these signals can be used to report on plant health. For person-to-plant communication, these signals can be initiated to drive plant resilience or novel responses. In the Woodson lab, we are also generating synthetic biology tools that can be used as genetic switches to control these signals.
CROPPS and the Woodson lab is currently interested in resolving three crucial questions about long distance signaling in plants:
1. How are long-distance signals initiated within the cell?
2. How is specific information encoded in long distance waves to allow for specific changes in plant resilience
3. Can synthetic biology tools be used to dissect these signals and generate stress tolerant varieties of crops?
We have been supported by the NSF Science and Technology Center CROPPS (Center for Research on Programmable Plants) grant DBI-2019674.
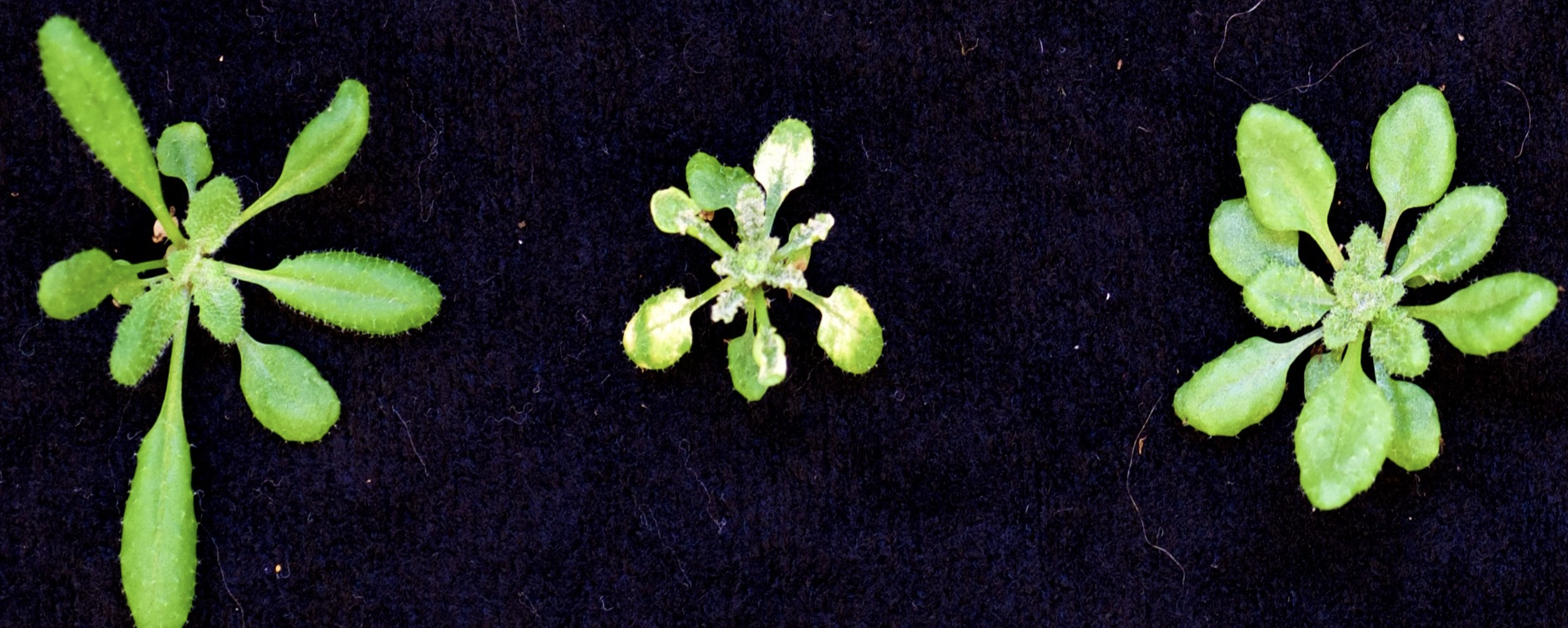